Department of Mechanical Engineering
INDIAN INSTITUTE OF TECHNOLOGY
(Banaras Hindu University)
“The task is...not so much to see what no one has yet seen; but to think what nobody has yet thought, about that which everybody sees.”
-Erwin Schrödinger
Hydrogen safety
Global warming has forced the world to shift away from Carbon-based fossil fuels. Hydrogen appears to be the preferred "green" fuel of choice. With growing international interest in Hydrogen-based systems, it becomes essential to consider the safety aspects of Hydrogen storage. I have developed a theoretical model to predict peak pressure attained in an accidental explosion. This model accounts for various realistic conditions like obstacles, stratified fuel, initial turbulence. This model is the first and only model that accounts for the flame front's fractal nature and how it affects spherical flame propagation. Predictions from this model are found to be more accurate than other models available in the literature. The proposed model is accepted for EN-14994, the statutory standard for Europe. Further efforts are underway to improve upon the prediction capabilities of this model. More details about this research can be found in the following papers:
https://doi.org/10.1016/j.ijhydene.2019.02.213
https://doi.org/10.1016/j.jlp.2019.05.017
Project details are given here:
https://cordis.europa.eu/project/id/671461
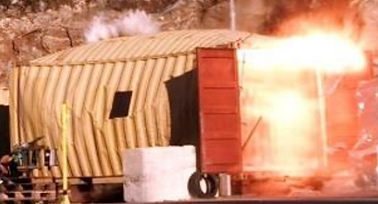
ISO container explosion at Gexcon facility
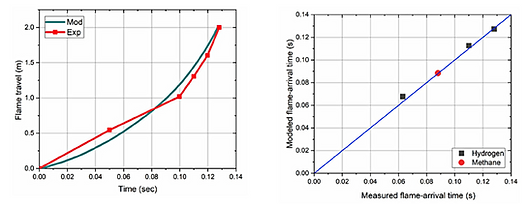
Flame arrival time predictions compared with experimental observation
Fuel injection



Liquid fuels are injected in spray, enabling them to mix and evaporate inside an engine or a gas turbine combustor. Detailed experiments help us understand the spray structure, dispersion, and mixing.
Liquid jet in crossflow (JIC) is a preferred strategy to inject fuel in gas turbine engines, afterburners, ramjets. Previous literature focuses mainly on the effect of operating conditions on jet behavior. The role of injector geometry is often overlooked. We have experimentally and theoretically investigated the role of injector geometry on jet surface instability and subsequent breakup. The theoretical analysis provides an equation to compute the wavelength of surface waves incorporating the effect of geometry and operating conditions. This equation matches well not only with our observations but also with data in the literature. Mode details are given in this paper:
https://doi.org/10.2514/1.J058383
We have also derived an equation for jet trajectory and reported droplet size distributions at various locations. That work is documented here:
https://doi.org/10.1016/j.expthermflusci.2017.12.012
After exploring jet in crossflow (JIC), we also examined spray in crossflow (SIC). In the case of SIC, we inject airblast spray instead of a liquid jet in JIC. For JIC, the primary atomization is caused by the aerodynamic force from the crossflow. On the other hand, for spray, the primary atomization is taken care of by the airblast gas inside the nozzle, and smaller droplets are injected. Further, we can control spray penetration in the crossflow by controlling the two-phase momentum ratio. We derived the trajectory equation for spray. Additionally, by using detailed droplet size and velocity measurements, we inferred the entrainment behavior of spray in crossflow and proposed a regime map for SIC.
https://doi.org/10.1016/j.ijmultiphaseflow.2015.02.008
https://doi.org/10.1007/s12046-019-1096-y
Further experiments were carried out in droplet evaporation. The evaporation behavior of multi-component polydisperse spray was studied under controlled conditions, and evaporation constants were derived.
https://doi.org/10.1016/j.fuel.2015.09.088
A numerical investigation was also carried out for SIC. The general assumption of considering perfect spherical droplets in numerical simulations is erroneous. A strategy to incorporate initial droplet distortion in the droplet breakup model was proposed.
https://doi.org/10.1177/1756827716652511

Spray dispersion - in context of COVID containment
Spray dispersion plays a critical role in transmitting and spreading airborne diseases such as SARS or COVID 19. A thorough understanding of how the spray from a cough or sneeze ejecta evolves with time and disperses in the surrounding is required to design control strategies for its containment. Hi-fidelity numerical simulations help us provide this critical insight and assess various strategies for aerosol containment. This study is one of the primary focuses of our current research, and we hope to contribute to the fight against the global pandemic. Details on experimental measurement of droplet sizes and velocities and spray modeling approaches can be found here:
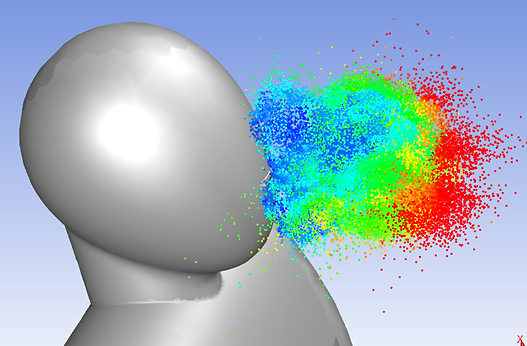
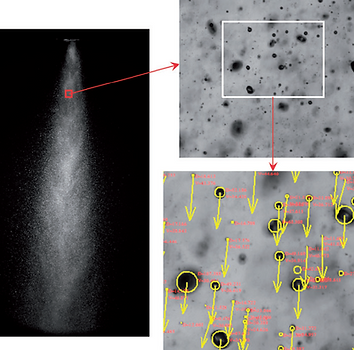
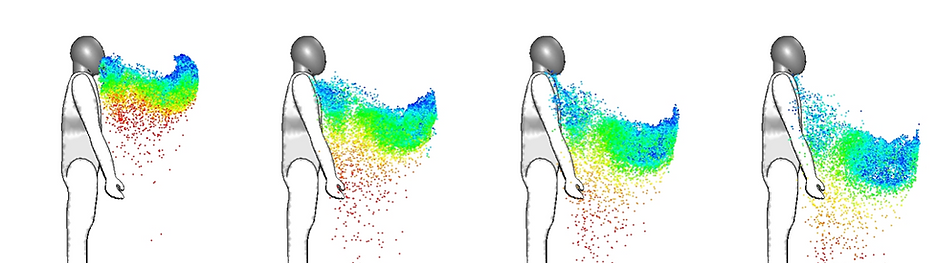
Flame stabilization
In aerospace applications, where the airspeed is much higher than the flame speed, providing a flame stabilizing mechanism to "hold" the flame becomes essential. One classic example is the bluff-body stabilized flame which is quite popular in devices such as afterburners. A detailed understanding of the recirculation zone, reaction kinetics, and heat transfer is required to design such systems. For such flame holder configuration, flame-wall interactions are also significant. Our group is actively working on projects that will help us understand the flame anchoring mechanisms and develop strategies to delay blowoff.
Recent advances have been made in other flame holding mechanisms, like Trapped Vortex Combustor (TVC). TVC follows a staged Rich burn-Quick mix-Lean burn (RQL) strategy, which significantly helps reduce emissions, particularly NOx. TVC and other advanced combustion concepts are also an area of interest where we try to improve combustor designs and improve engine performance and operability.

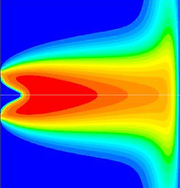
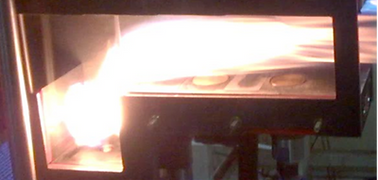

Modal decomposition of fluid flows
Modal decomposition techniques such as Proper Orthogonal Decomposition (POD) and Dynamic Mode Decomposition (DMD) can be used to identify dynamic flow features from the flow field data. These techniques are increasingly becoming popular owing to their ability to extract useful information from instantaneous snapshots and their application in Machine learning.
Our group explores modal decomposition techniques on various experimental and computational data sets and extracts and analyzes flow field information. We hope to create a framework for the use of Machine learning in pattern recognition and to be able to predict the temporal evolution of turbulent and reacting flows.

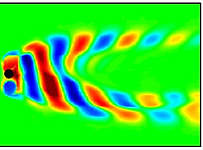
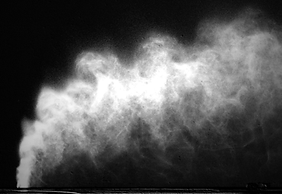
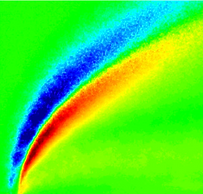
